HUMAN NEUROBIOLOGY
THE NEURON
The
functional unit of the nervous system is the neuron, a term
coined by Waldeyer in 1891. Neurons are the excitable cells of
nervous tissue which conduct impulses. They arise from the
neuroblasts of neural tube and neural crest origin. The complex
nervous systems of vertebrates, especially humans, represent
remarkably coordinated networks of these fundamental units. Thus
it is not surprising to find great diversity in the form and
function of individual neurons. Consequently, neurons are
classified according to a number of different criteria: (1)
morphology or appearance, (2) anatomical location, (3) whether
they are sensory or motor, (4) conduction velocity, (5) fiber
diameter, and (6) whether they are myelinated or not.
Morphological Classification
Neurons are
single cells composed of a perikaryon or cell body (soma) and a
variable number of neurites (processes) extending out from it.
Adult neurons are classified as monopolar, bipolar, or
multipolar according to whether the perikaryon has one, two, or
many neurites (Fig-1).
Monopolar
Neurons
Monopolar neurons have only one prominent neurite
extending from the perikaryon, which then branches into two long
processes, one central (directed toward the CNS) and one
peripheral (directed away from the CNS). Most neurons of this
type are sensory and are exclusively located in the peripheral
nervous system. The dorsal root ganglion cells of the spinal
nerves are monopolar neurons. They relay information from
receptors sensitive to touch, pressure, pain, temperature, and
stretch, as well as body position and movement.
Bipolar
Neurons
Bipolar neurons have two prominent neurites extending
from the perikaryon. One conducts impulses toward and one away
from the soma. Bipolar neurons are found in the retina, the
cochlear and vestibular ganglia, the olfactory epithelium, and
in some parts of the central nervous system.
Multipolar
Neurons
These are by far the most common type of neuron. They
populate both the central and peripheral nervous systems and are
characterized by several short, highly branched processes
called dendrites and a single, long process extending out from
the soma called an axon. A slight enlargement at the point
where the axon leaves the soma, called the axon hillock, is
often observed.
Considerable confusion exists regarding the proper use of the
terms dendrite and axon. A workable definition is that dendrites
are the processes which are specialized to receive stimuli from
other cells, while the axon is specialized to conduct impulses.
This applies quite adequately to multipolar neurons, but the
terms are arbitrary and confusing when applied to monopolar and
bipolar neurons. Both the central and peripheral processes of
the latter two types conduct impulses. Some neuroscientists
refer to both as axons while others call the central process an
axon and the peripheral process a dendrite.
A system
proposed by Bodian describes that portion of the neuron which is
specialized to receive stimuli from other neurons or receptors
as the dendritic zone. He further describes that portion which
is specialized to conduct impulses (essentially the rest of the
neuron) as the axon. Accordingly, the soma is included in the
dendritic zone of multipolar neuron because a considerable
number of synapses (contacts) from other neurons converge on it.
However, no synaptic contacts are made with the somas of
monopolar and bipolar neurons. In fact, only a very limited part
of one of the processes of these latter two types actually
receives synaptic contacts from other neurons or receptors. Thus
this limited area represents the dendritic zone of monopolar and
bipolar neurons. Impulses generated here are then conducted over
the rest of the neuron, including the soma. In Bodian's system
all of this impulse-conducting portion is the axon. The
dendritic zones and axons of the three types of neurons are
illustrated in Fig-2.
|
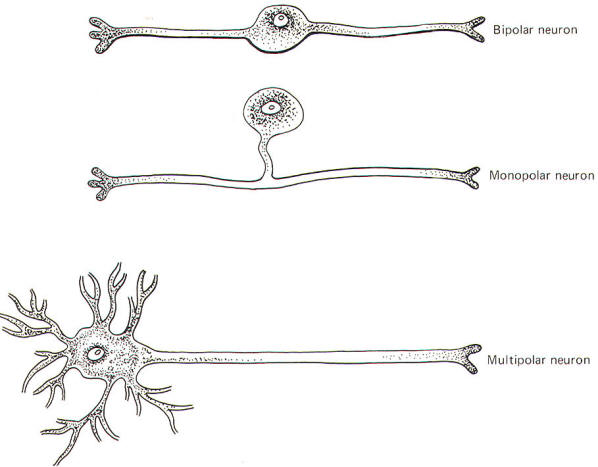 |
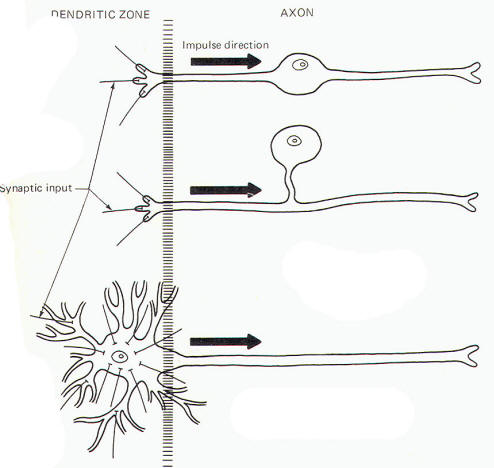 |
|
|
|
Fig-1 |
Fig-2 |
|
|
Classification of Nerve Fibers by Group and Type
A neuron is
afferent to a particular site if it conducts impulses toward it
and efferent from that site if it conducts impulses away. For
example, a neuron which conducts impulses from the thalamus to
the cerebral cortex is efferent from the thalamus and afferent
to the cerebral cortex.
An efferent
neuron which directly innervates a muscle or a gland and causes
it to respond in some way is called a motor neuron. An afferent
neuron which responds to changes in the external or internal
environment and gives rise to conscious sensation is termed a
sensory neuron. The latter is a strict definition of the term sensory. Not all afferent neurons give rise to conscious
sensation, and thus not all afferent neurons are sensory.
Nevertheless, the two terms (sensory and afferent) are often
used interchangeably.
Historically, mammalian PNS nerve fibers can be classified by
group or type because of an observed correlation between
conduction velocity and fiber diameter. The group system
classifies afferent fibers only, while the type system
classifies both. The two systems are codified in Table-1.
Table-1
Group and Type Classification of Mammalian Nerve Fibers |
Group |
Type |
Fiber diameter µm |
Conduction velocity m/s |
Description |
la |
A
|
13-22 |
70-120 |
Alpha motor neurons to
skeletal muscles |
A
|
13-22 |
70-120 |
Primary afferents from
muscle spindles |
Ib |
A |
13-22 |
70-120 |
Afferents from Golgi
tendon organs |
II |
A
|
8-13 |
40-70 |
Secondary afferents from
muscle spindles, afferents
from touch and pressure
receptors |
A |
4-8 |
15-40 |
Gamma motor neurons to
muscle spindles |
III |
A |
1-5 |
5-15 |
Afferents from touch,
pressure, pain, and
temperature receptors |
B
|
0.1-3 |
0.3-14 |
Preganglionic autonomic fibers |
C |
0.1-3 |
0.2-2 |
Postganglionic autonomic fibers |
IV |
C
|
0.1-3 |
0.2-2 |
Afferents from pain and
temperature receptors |
Nerves and
Nerve Fiber Tracts
The long
process which extends out from the soma of the nerve cell is
also called a nerve fiber. These fibers are distributed
throughout the peripheral nervous system in anatomically
distinct structures called nerves. It is important to note that
nerves exist only in the peripheral nervous system. There are no
nerves within the brain or spinal cord itself. Instead, nerve
fibers are distributed throughout the central nervous system in
reasonably distinct anatomical groupings called nerve fiber
tracts. Thus it is appropriate to speak of a spinal or cranial
nerve since it is part of the peripheral nervous system but not
to speak of a nerve within the brain or spinal cord. Tract is
the appropriate terminology here. An example is the anterior
spinothalamic tract, which is composed of a group of fibers
which conduct impulses from the spinal cord to the thalamus, a
route entirely within the CNS.
Schwann
Cells
The Schwann
cells are the nonexcitable cells of the peripheral nervous
system. By definition they do not conduct impulses. Recall that
Schwann cells are derived from Schwann cell precursors of the
primitive neural crests. They develop in close association with
all of the neuroblasts of the peripheral nervous system. In some
cases this association is so close that the Schwann cells wrap
many times around the axon of a developing neuron, laying down
layer after layer of myelin and producing a myelinated neuron.
In other cases, the association is not characterized by
wrapping Schwann cells and the neuron remains non myelinated.
Myelinated
Neurons In those neurons destined to become myelinated, a
Schwann cell begins to wrap around a given length of axon in a
spiral fashion. In doing
so, the Schwann cell extrudes its cytoplasm as its two membranes
press together. In this manner it lays down layer after layer of
its own membrane, forming a laminated sheath of highly lipid
material called myelin. Several Schwann cells myelinate a single
axon in this manner, each on a different section of its length.
Because of
the tight packing and lamination of the myelin, the small
volume of fluid in the periaxonal space immediately surrounding
the axon (Figs-3 and 4) is not readily interchangeable with
the extracellular fluid of the nerve trunk. The external and
internal mesaxons formed by the circling Schwann cell are not
free conduits for fluid exchange. The mesaxon is the double
membrane formed by the Schwann cell. Thus the axonal membrane is
only in contact with a freely interchangeable fluid space at the
node of Ranvier, where one Schwann cell meets another. The
unique anatomical arrangement of Schwann cells around the axons
of myelinated neurons endows them with a special pattern of
impulse conduction called saltatory conduction. The entire
Schwann cell is surrounded by a basement membrane, which
together with the outer Schwann cell membrane comprises the
neurilemma.
|
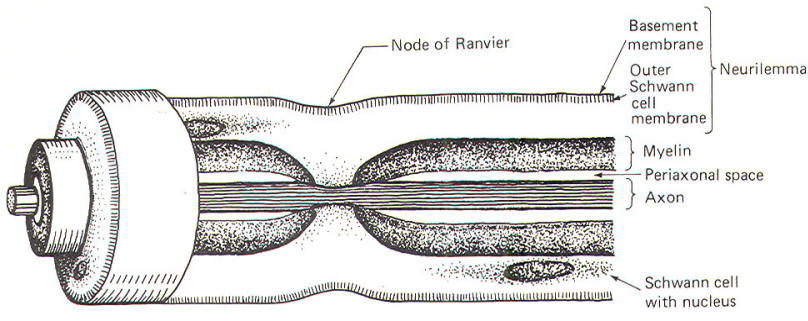 |
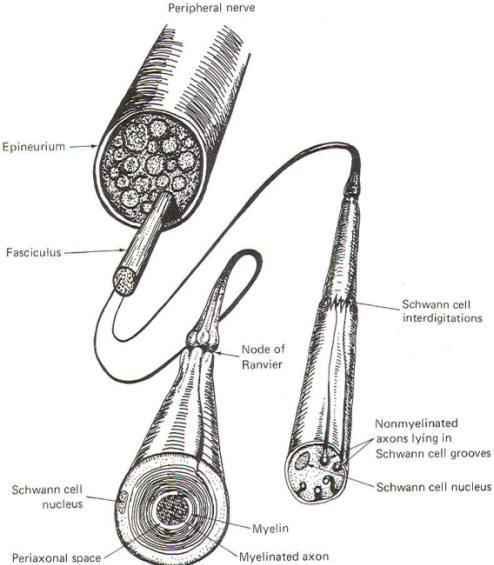 |
|
|
Fig-3 |
Fig-4 |
|
Nonmyelinated Neurons Postganglionic autonomic fibers as well as
some of the very narrow diameter nerve fibers from pain and
temperature receptors form a
rather loose relationship with Schwann cells. These type C nerve
fibers are usually found running in long, deep longitudinal
depressions in Schwann cells (Fig-4). A single Schwann cell
may have depressions for several narrow fibers. In this case,
unlike myelinated axons, the extracellular fluid of the nerve
trunk is in contact with the axonal membrane via a gap in the mesaxon which is continuous with the periaxonal space. Thus the
entire non myelinated axon is in constant contact with a freely
interchangeable fluid space and the pattern of impulse
conduction is therefore not saltatory as observed in myelinated
axons. Even though Schwann cells are in intimate contact with
these axons, they are not myelinated because they have not been
wrapped by the cells. The characteristics of saltatory and
nonsaltatory conduction will be explained in detail elsewhere.
As Fig-4 shows, Schwann cells form loose contacts with nonmyelinated axons and several of the cells interdigitate with
each other enveloping the axon throughout its length.
Neuroglial
Cells
Neuroglia
("neural glue") is a fine web of tissue which is composed of
peculiar branched cells called neuroglial cells. They are
located in the central nervous system only and fall into two
categories: macroglia and microglia. Macroglial cells are
derived from glioblasts of the neural tube and include small
starshaped cells called astrocytes as well as oligodendrocytes,
which are the CNS equivalent of Schwann cells. Microgliocytes
are small nonneural cells, possibly of mesodermal origin.
Neuroglial
cells playa variety of roles in the CNS. Astrocytes appear to
influence the transport of materials to the neurons of the
central nervous system as well as to function to maintain an
appropriate ionic environment for the neurons.
Oligodendrocytes are responsible for myelinating the neurons of
the central nervous system. However, unlike a single Schwann
cell, which can only myelinate a single axon, each
oligodendrocyte can myelinate the axons of several CNS neurons.
As previously mentioned, microgliocytes are probably not of CNS
origin at all. They are small cells of various forms with
slender, branched processes which migrate into the CNS and act
as phagocytes scavenging for waste products and breakdown
components of CNS neurons.
Ependymal
Cells
Recall that
the ependyma is a single layer of epithelial cells lining the
ventricles of the brain and the central canal of the spinal
cord. They arise from the fixed neuroepithelial cells lining
the neural tube. Later they differentiate into the ependymal
linings of the central nervous system.
CENTRAL
NERVOUS SYSTEM
Neuroscientists define the central nervous system as the brain
and spinal cord. The brain is considered to include the cerebral
hemispheres, brainstem, and cerebellum. The brainstem includes
the diencephalon, midbrain, pons, and medulla oblongata.
The Brain
Several
surface features of the brain are illustrated in Fig-5. When
the meningeal coverings are removed it is apparent that the
cerebrum is divided into two equal hemispheres by a deep median,
longitudinal fissure. It is also apparent that the surface of
each hemisphere is very irregular with many ridges (gyri)
separated by shallow grooves (sulci). A particularly deep sulcus
is called e fissure. A central sulcus separates each hemisphere
into a frontal (anterior) lobe and a parietal (posterior) lobe.
A temporal lobe is separated from the frontal lobe in each
hemisphere by a lateral fissure. The occipital lobe in each
hemisphere is marked off by the parietooccipital fissure and
the preoccipital notch.
Additional
features of the anterior lobe are the superior, middle, and
inferior frontal gyri and sulci. Just anterior to the central
sulcus is the precentral sulcus and gyrus, while just posterior
to it in the parietal lobe is the postcentral gyrus and sulcus.
Each temporal lobe is characterized by a superior, middle, and
inferior temporal gyrus and sulcus.
Several
additional brain features can be seen in the median sagittal
section illustrated in Fig-6. The cingulate gyrus is a
primitive band of cortical tissue circling the corpus callosum.
The latter is a thick band of commissural (connecting) fibers
between the two cerebral hemispheres. The septum pellucidum is a
thin membrane separating the cerebrospinal fluid of the two
lateral hemispheres. It can be seen between the fornix and the
anterior portion of the corpus callosum.
The medial
surfaces of the thalamus and hypothalamus form the lateral walls
of the third ventricle, which is continuous with the lateral
ventricles above through the foramina of Monro and with the
fourth ventricle below through the cerebral aqueduct. The
anterior and posterior comrnissures, like the corpus callosum,
are bands of fibers which connect the two hemispheres. The
pineal body and colliculi are prominent features of the
posterior brainstem, while the optic chiasm, pituitary gland,
and mammillary bodies are prominent anterior features.
|
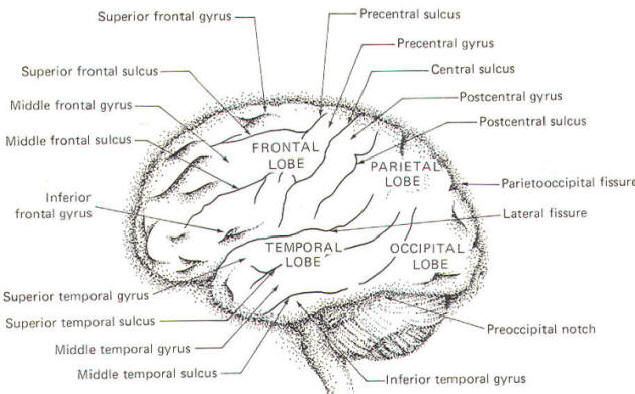 |
|
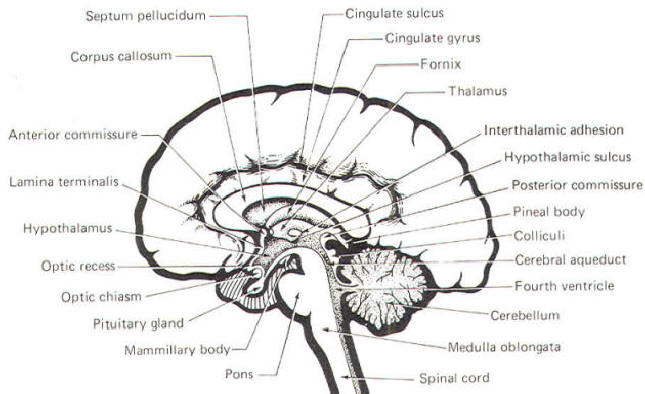 |
|
Fig-5 |
|
Fig-6 |
The Spinal
Cord
The spinal
cord is the caudal extension of the brain stem into the
vertebral canal. It is essentially a long, narrow structure with
a cervical and lumbar enlargement. The cervical enlargement is
due to the great number of afferent and efferent spinal nerve
fibers from this region which innervate the arms. The lumbar
enlargement represents a similar innervation of the leg
musculature.
Several
prominent sulci are noticeable in a posterior view of the cord
(Fig-7). These include a single posterior median sulcus with
posterior intermediate and posterior lateral sulci on either
side of it. An anterior view shows an anterior median fissure
with an anterior lateral sulcus on either side. A long, thin extension of the spinal cord, the filum terminale, extends to
the coccyx at the tip of the sacrum. A cross section of the
spinal cord at any level will show the characteristic
butterfly-shaped pattern of gray matter surrounded by white
matter. In Fig-8 notice that the relative amount of gray
matter to white matter varies from one level of the cord to
another.
Spinal Cord
White Matter
The spinal
cord white matter is divided into three large regions called
funiculi. The posterior funiculus is bounded by the posterior
median and posterior lateral sulci (Fig-9). The lateral
funiculus is that region of white matter between the posterior
lateral and anterior lateral sulci. The anterior funiculus is
bounded by the anterior lateral sulcus and the anterior median
fissure. The white matter on both sides of the cord is
continuous through the anterior white commissure.
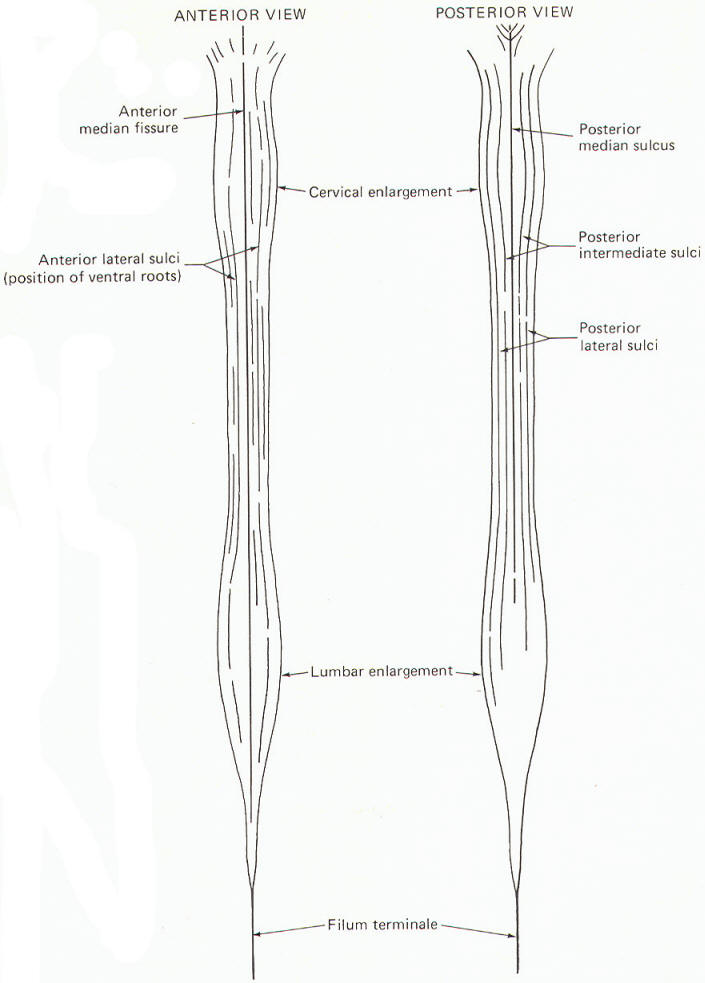 |
|
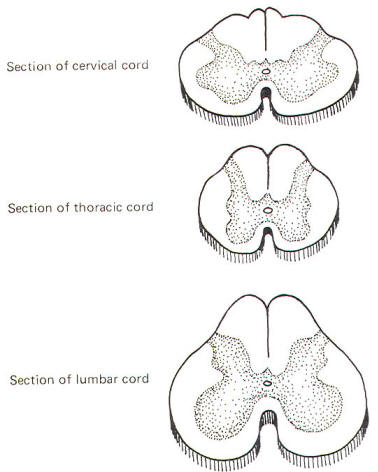 |
|
|
Fig-8 |
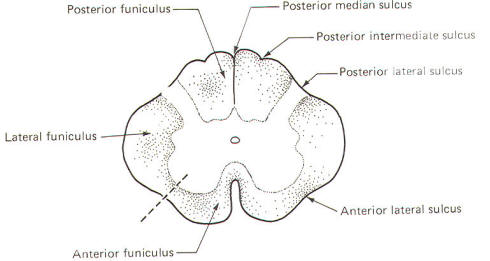 |
Fig-9 |
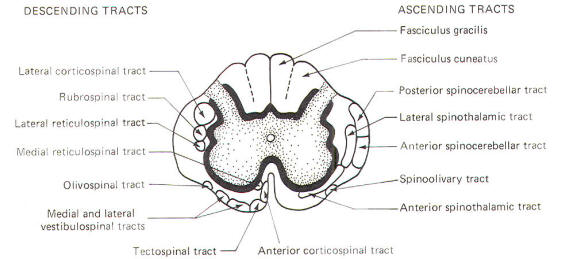 |
Fig-10 |
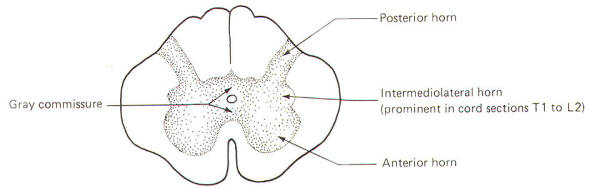 |
Fig-7 |
|
Fig-11 |
|
|
Ascending
and Descending Tracts in the Spinal Cord White Matter
The spinal
cord white matter is composed of millions of ascending and
descending fibers. The ascending fibers conduct impulses up the
cord while descending fibers conduct impulses downward. Most of
these fibers have also been myelinated by oligodendrocytes, and
it is their resulting myelin sheaths which give the white matter
its characteristic color.
Most of the
spinal cord fibers are grouped together in functional units
called tracts. The descending tracts typically become smaller as
they pass downward through the cord. This is caused by fibers
continually leaving the tracts as they reach their specific
destinations. Ascending tracts,
with each tract associated with a functional role. The addition
of a functional component as well as an examination of the
clinical signs associated with selective destruction of the
various tracts will make it easier to comprehend the anatomical
distribution of the tracts illustrated in Fig-10.
Spinal Cord
Gray Matter
The gray
matter in each half of the cord is subdivided into a posterior,
intermediolateral, and anterior horn. The gray commissure
connects the gray matter on each side of the cord around the
central canal (Fig-11).
Recognize
that Fig-11 is also a composite. Comparison with Fig-8
will help to clarify what a composite is. Because the anterior
horn contains the cell bodies of motor neurons to the skeletal
muscles, it is considerably larger in the cervical and lumbar
enlargements where the cord gives rise to the spinal nerves
innervating the arms and legs. Also, because most of the sensory
fibers of spinal nerves terminate in the posterior horn, it is
not surprising to find a larger horn in the cervical and lumbar
regions than in the thoracic cord.
An
intermediolateral horn, which gives rise to preganglionic
sympathetic neurons, is found only in cross sections of the cord
between T1 and L2. A similar region giving rise to
preganglionic parasympathetic neurons is located in the
intermediate gray matter of sacral cord segments 2 to 4.
However, unlike segments T1 through L2, it does not extend as a
noticeable lateral "horn."
Laminar
Architecture of the Gray Matter
A
convenient way to subdivide the gray matter of the spinal cord
is according to the general cytoarchitecture found in its
various regions. These cell regions, or laminae, are illustrated
in Fig-12. It should be noted that this scheme is based on
the spinal cord of the cat. Nevertheless, the system is being
applied with due caution to humans.
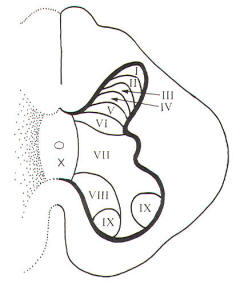 |
Fig-12 |
Laminae I,
II, III, and IV are thought to be the principal sensory
receiving areas for afferent input to the cord. Laminae V and VI
deal with proprioceptive input (dealing with body position and
movement) as well as input from the cerebral cortex and other
higher centers. Lamina VII has connections with many higher
centers. Lamina VIII receives input from the opposite side of
the cord as well as having numerous connections with higher
brain centers. Lamina IX is the region of alpha and gamma motor
neurons to skeletal muscles. Lamina X is probably a commissural
area. It cannot be overstressed that the suspected roles
assigned to the laminae above represent a considerable
oversimplification. Nevertheless, it gives a basis for
understanding synaptic relays as they relate to ascending and
descending tracts in the cord and afferent input and efferent
output with spinal nerves.
PERIPHERAL
NERVOUS SYSTEM
The
peripheral nervous system is composed of 12 pairs of cranial
nerves and 31 pairs of spinal nerves. It represents an extension
of the central nervous system into the far reaches of the body.
Each spinal nerve contains both afferent and efferent fibers
while cranial nerves, on the other hand, are more diverse. Some
are afferent only, some are efferent only, and some are mixed
(both afferent and efferent).
Spinal
Nerves
Each pair
of spinal nerves extends laterally from the cord at regular
intervals from cervical to coccygeal regions. The spinal nerves
leave the vertebral canal via regular openings in the vertebral
column called intervertebral foramina (Fig-14). Each spinal
nerve is named according to the intervertebral foramina through
which it exits. Notice in Fig-13 that there is one spinal
nerve pair for each vertebra with the exception of the cervical
region, where there are seven cervical vertebrae but eight
cervical nerve pairs.
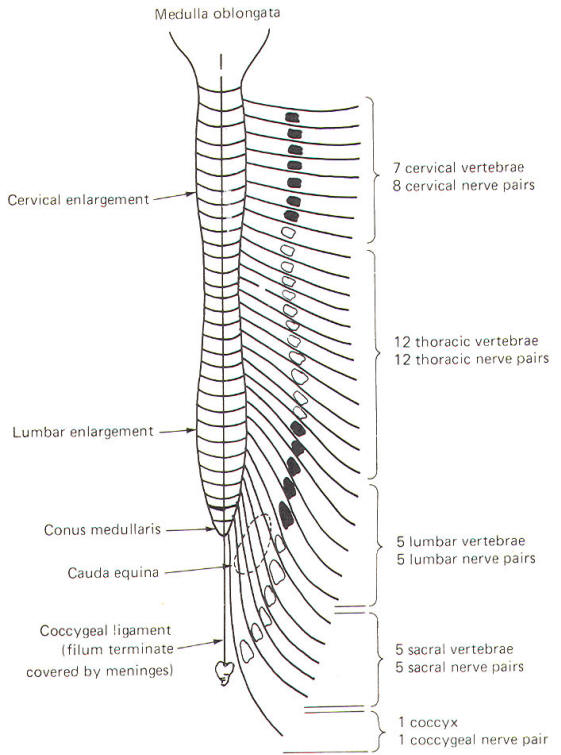 |
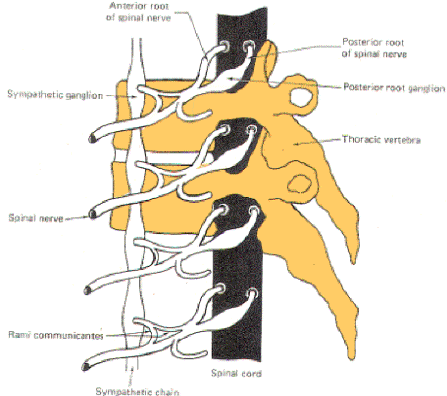 |
Fig-13 |
Fig-14 |
Each spinal
nerve communicates with the spinal cord via two short roots, one
posterior (dorsal) and one anterior (ventral). The anterior and
posterior roots of the spinal nerves lie entirely within the
vertebral canal. They join and form the spinal nerve just before
it exits the vertebral canal through the intervertebral
foramen.
At the
point where spinal nerves T1 through L2 leave their respective
foramina, two short arms (rami communicantes) connect the spinal
nerve with a sympathetic ganglion lying adjacent to the body of
the vertebra (Fig-14). A ganglion is a group of cell bodies
located outside of the CNS. These 14 ganglia are connected to
each other to form a vertical sympathetic chain. Three
additional ganglia, the superior, middle, and inferior
cervical, join the chain superiorly while another three to five
ganglia in the lumbar region join it inferiorly. Thus 20 to 22
ganglia make up the sympathetic chain on each side of the cord.
The three cervical ganglia and the last three to five ganglia in
the chain communicate with spinal nerves via only one ramus
while the ganglia associated with spinal nerves T1 through L2
communicate through two.
Cranial
Nerves
There are
12 pairs of cranial nerves communicating directly with the
brain. They are analogous with the 3 1 pairs of spinal nerves
which communicate with the spinal cord. They include the
following:
I
|
Olfactory |
VII
|
Facial |
II
|
Optic |
VIII |
Vestibulocochlear |
III
|
Oculomotor |
IX
|
Glossopharyngeal |
IV
|
Trochlear |
X
|
Vagus |
V
|
Trigeminal |
XI
|
Accessory |
VI
|
AbducCNS |
XII
|
Hypoglossal |
Cranial
Nerve Emergence and the Brainstem
The cranial
nerves and their relationship to the brain stem are shown in
Fig-15. The olfactory nerves (I) enter through the cribiform
plate of the ethmoid bone as 20 to 30 fila (threadlike
structures) to contact the olfactory bulb. The optic nerves (II)
cross anterior to the pituitary gland in the optic chiasm and
continue around the cerebral peduncles of the midbrain as the
optic tracts. The oculomotor nerves (III) originate in the
midbrain and emerge anteriorly close together at the superior
border of the pons. The trochlear nerves (IV) emerge from the
posterior surface of the midbrain just below the inferior
colliculi. From here they wrap around the cerebral peduncles to
appear anteriorly at the superior border of the pons. The
trigeminal nerves (V) arise from the anterolateral surface of
the pons. The abducens nerves (VI) originate in the pons and
emerge close together at the anterior inferior border of the
pons. The facial nerves (VII). originating in the pons. and the
vestibulocochlear nerves (VIII), which originate in the upper
medulla. emerge laterally at the pontomedullary border.
Emerging
from the lateral medulla posterior to the olive (a rounded
elevation lateral to the pyramids in the medulla oblongata) in
order from superior to inferior are the glossopharyngeal nerves
(IX), vagus nerves (X), and bulbar accessory nerves (XI).
Appearing with them but originating in the spinal accessory
nuclei of the upper cervical cord are the spinal portions of the
accessory nerves. the spinal accessory nerves (XI). Finally,
arising from the lateral medulla anterior to the olive are the
hypoglossal nerves (XII).
CLASSIFICATION OF SPINAL AND CRANIAL NERVE FIBERS
Spinal
nerve fibers are either afferent or efferent with respect to the
spinal cord (Table-2). The spinal efferent fibers are either
somatic (innervating skeletal muscles derived from mesodermal somites) or visceral (innervating cardiac muscle, smooth muscle,
or glands). Similarly, spinal afferent fibers are also
classified as either somatic or visceral. All are called general
fibers.
Cranial
nerve fibers are classified in the same manner as spinal nerve
fibers (Table-2). However, there is also a special
classification. Nerve fibers classified as special innervate
the special sense organs involved in hearing, seeing, smelling,
and tasting. In addition, special fibers innervate the
vestibular system and those skeletal muscles derived from the
mesoderm of the branchial arches (embryonic segments which give
rise to structures in the ear and neck).
Table-2
Classification of Spinal and Cranial Nerve Fibers |
I |
Spinal
nerve fiber classification |
|
A |
|
General afferent fibers. |
The afferent unipolar neurons of the posterior
root of the spinal cord
with cell bodies in the posterior root ganglia |
|
|
1 |
General somatic afferent (GSA) |
From exteroceptors
responding to touch, pressure, pain, and temperature as well as
from the proprioceptors of muscles, tendons, and joints
|
|
|
2 |
General visceral afferent (GVA) |
From interoceptors of the viscera |
|
B |
|
General
efferent fibers.
|
The efferent multipolar neurons with cell
bodies in the anterior and intermediolateral horns of the spinal
cord gray matter |
|
|
1 |
General somatic efferent (GSE) |
The alpha and gamma motor neurons to
somatic skeletal muscle and muscle spindles with cell
bodies in the anterior horn of the spinal cord gray
matter |
|
|
2 |
General visceral efferent (GVE) |
The autonomic fibers to cardiac muscle,
smooth muscle, and
glands |
II |
Cranial nerve fiber classification |
|
A |
|
General afferent fibers. |
The afferent unipolar neurons with cell bodies
in the craniospinal
ganglia |
|
|
1 |
General somatic afferent (GSA). |
From exteroceptors responding to touch, pressure, pain, and
temperature as well as from the proprioceptors of muscles,
tendons, and joints |
|
|
2 |
General visceral afferent (GVA). |
From interoceptors of the viscera |
|
B |
|
Special afferent fibers. |
The afferent neurons from the special sense organs (eye, ear, nose, and tongue) and the vestibular
system |
|
|
1 |
Special somatic afferent (SSA). |
Exteroceptors
from the eye and ear as well as proprioceptors from the
vestibular system |
|
|
2 |
Special
visceral afferent (SVA). |
Exteroceptors from the olfactory
epithelium and the taste
buds |
|
C |
|
General efferent fibers. |
The efferent neurons originating in brainstem
nuclei innervating somatic skeletal muscle as well as those
innervating cardiac muscle, smooth muscle, and glands
|
|
|
1 |
General somatic efferent (GSE). |
To somatic skeletal muscles |
|
|
2 |
General visceral efferent (GVE). |
The autonomic fibers to cardiac muscle,
smooth muscle, and
glands |
|
D |
|
Special efferent fibers. |
The efferent neurons originating in brainstem
nuclei innervating
branchiomeric skeletal muscle |
|
|
1 |
Special
visceral efferent (SVE). |
To branchiomeric skeletal muscles |
|